Top Qs
Timeline
Chat
Perspective
Irrationality measure
Function that quantifies how near a number is to being rational From Wikipedia, the free encyclopedia
Remove ads
In mathematics, an irrationality measure of a real number is a measure of how "closely" it can be approximated by rationals.
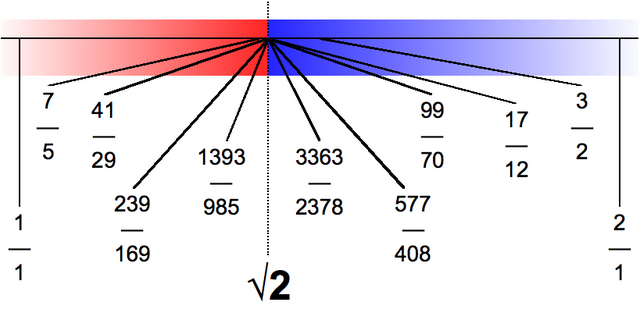
If a function , defined for , takes positive real values and is strictly decreasing in both variables, consider the following inequality:
for a given real number and rational numbers with . Define as the set of all for which only finitely many exist, such that the inequality is satisfied. Then is called an irrationality measure of with regard to If there is no such and the set is empty, is said to have infinite irrationality measure .
Consequently, the inequality
has at most only finitely many solutions for all .[1]
Remove ads
Irrationality exponent
Summarize
Perspective
The irrationality exponent or Liouville–Roth irrationality measure is given by setting ,[1] a definition adapting the one of Liouville numbers — the irrationality exponent is defined for real numbers to be the supremum of the set of such that is satisfied by an infinite number of coprime integer pairs with .[2][3]: 246
For any value , the infinite set of all rationals satisfying the above inequality yields good approximations of . Conversely, if , then there are at most finitely many coprime with that satisfy the inequality.
For example, whenever a rational approximation with yields exact decimal digits, then
for any , except for at most a finite number of "lucky" pairs .
A number with irrationality exponent is called a diophantine number,[4] while numbers with are called Liouville numbers.
Corollaries
Rational numbers have irrationality exponent 1, while (as a consequence of Dirichlet's approximation theorem) every irrational number has irrationality exponent at least 2.
On the other hand, an application of Borel-Cantelli lemma shows that almost all numbers, including all algebraic irrational numbers, have an irrationality exponent exactly equal to 2.[3]: 246
It is for real numbers and rational numbers and . If for some we have , then it follows .[5]: 368
For a real number given by its simple continued fraction expansion with convergents it holds:[1]
If we have and for some positive real numbers , then we can establish an upper bound for the irrationality exponent of by:[6][7]
Known bounds
For most transcendental numbers, the exact value of their irrationality exponent is not known.[5] Below is a table of known upper and lower bounds.
Remove ads
Irrationality base
The irrationality base or Sondow irrationality measure is obtained by setting .[1][6] It is a weaker irrationality measure, being able to distinguish how well different Liouville numbers can be approximated, but yielding for all other real numbers:
Let be an irrational number. If there exist real numbers with the property that for any , there is a positive integer such that
for all integers with then the least such is called the irrationality base of and is represented as .
If no such exists, then and is called a super Liouville number.
If a real number is given by its simple continued fraction expansion with convergents then it holds:
- .[1]
Examples
Any real number with finite irrationality exponent has irrationality base , while any number with irrationality base has irrationality exponent and is a Liouville number.
The number has irrationality exponent and irrationality base .
The numbers ( represents tetration, ) have irrationality base .
The number has irrationality base , hence it is a super Liouville number.
Although it is not known whether or not is a Liouville number,[32]: 20 it is known that .[5]: 371
Remove ads
Other irrationality measures
Markov constant
Setting gives a stronger irrationality measure: the Markov constant . For an irrational number it is the factor by which Dirichlet's approximation theorem can be improved for . Namely if is a positive real number, then the inequality
has infinitely many solutions . If there are at most finitely many solutions.
Dirichlet's approximation theorem implies and Hurwitz's theorem gives both for irrational .[33]
This is in fact the best general lower bound since the golden ratio gives . It is also .
Given by its simple continued fraction expansion, one may obtain:[34]
Bounds for the Markov constant of can also be given by with .[35] This implies that if and only if is not bounded and in particular if is a quadratic irrational number. A further consequence is .
Any number with or has an unbounded simple continued fraction and hence .
Other results
The values and imply that the inequality has for all infinitely many solutions while the inequality has for all only at most finitely many solutions . This gives rise to the question what the best upper bound is. The answer is given by:[36]
which is satisfied by infinitely many for but not for .
This makes the number alongside the rationals and quadratic irrationals an exception to the fact that for almost all real numbers the inequality below has infinitely many solutions :[5] (see Khinchin's theorem)
Remove ads
Mahler's generalization
Summarize
Perspective
Kurt Mahler extended the concept of an irrationality measure and defined a so-called transcendence measure, drawing on the idea of a Liouville number and partitioning the transcendental numbers into three distinct classes.[3]
Mahler's irrationality measure
Instead of taking for a given real number the difference with , one may instead focus on term with and with . Consider the following inequality:
with and .
Define as the set of all for which infinitely many solutions exist, such that the inequality is satisfied. Then is Mahler's irrationality measure. It gives for rational numbers, for algebraic irrational numbers and in general , where denotes the irrationality exponent.
Transcendence measure
Mahler's irrationality measure can be generalized as follows:[2][3] Take to be a polynomial with and integer coefficients . Then define a height function and consider for complex numbers the inequality:
with .
Set to be the set of all for which infinitely many such polynomials exist, that keep the inequality satisfied. Further define for all with being the above irrationality measure, being a non-quadraticity measure, etc.
Then Mahler's transcendence measure is given by:
The transcendental numbers can now be divided into the following three classes:
If for all the value of is finite and is finite as well, is called an S-number (of type ).
If for all the value of is finite but is infinite, is called an T-number.
If there exists a smallest positive integer such that for all the are infinite, is called an U-number (of degree ).
The number is algebraic (and called an A-number) if and only if .
Almost all numbers are S-numbers. In fact, almost all real numbers give while almost all complex numbers give .[37]: 86 The number e is an S-number with . The number π is either an S- or T-number.[37]: 86 The U-numbers are a set of measure 0 but still uncountable.[38] They contain the Liouville numbers which are exactly the U-numbers of degree one.
Linear independence measure
Another generalization of Mahler's irrationality measure gives a linear independence measure.[2][13] For real numbers consider the inequality
with and .
Define as the set of all for which infinitely many solutions exist, such that the inequality is satisfied. Then is the linear independence measure.
If the are linearly dependent over then .
If are linearly independent algebraic numbers over then .[32]
It is further .
Remove ads
Other generalizations
Summarize
Perspective
Koksma’s generalization
Jurjen Koksma in 1939 proposed another generalization, similar to that of Mahler, based on approximations of complex numbers by algebraic numbers.[3][37]
For a given complex number consider algebraic numbers of degree at most . Define a height function , where is the characteristic polynomial of and consider the inequality:
with .
Set to be the set of all for which infinitely many such algebraic numbers exist, that keep the inequality satisfied. Further define for all with being an irrationality measure, being a non-quadraticity measure,[17] etc.
Then Koksma's transcendence measure is given by:
- .
The complex numbers can now once again be partitioned into four classes A*, S*, T* and U*. However it turns out that these classes are equivalent to the ones given by Mahler in the sense that they produce exactly the same partition.[37]: 87
Simultaneous approximation of real numbers
Given a real number , an irrationality measure of quantifies how well it can be approximated by rational numbers with denominator . If is taken to be an algebraic number that is also irrational one may obtain that the inequality
has only at most finitely many solutions for . This is known as Roth's theorem.
This can be generalized: Given a set of real numbers one can quantify how well they can be approximated simultaneously by rational numbers with the same denominator . If the are taken to be algebraic numbers, such that are linearly independent over the rational numbers it follows that the inequalities
have only at most finitely many solutions for . This result is due to Wolfgang M. Schmidt.[39][40]
Remove ads
See also
References
Wikiwand - on
Seamless Wikipedia browsing. On steroids.
Remove ads